[Maschinenhaus]
Active member
Chapter 1
There are 6 important factors that can affect cannabis growth and flowering: nutrients, water supply, light intensity, oxygen and CO².
Nutrients:
Most of the building blocks of plants are present in the atmosphere. Fertilization affects growth by only about 6 percent. Therefore, do not use more fertilizer than necessary, as this could affect growth. The root can only absorb nutrient salts as ions dissolved in the water. For this reason, the soil should be sufficiently moist to allow the uptake of mineral fertilizers and compounds mineralized by soil creatures. The water in the soil splits the fertilizer salts into ions. For example, nitrate of lime (Ca(NO3)2) breaks down into the positively charged calcium ion (Ca2+) and two negatively charged nitrate ions (NO3-).
The nutrient salt ions are found in soil in three forms:
- as freely mobile ions dissolved in the water (very easily absorbable)
- interchangeably bound to negatively charged clay and humus particles (relatively easy to absorb)
- as reserve nutrients in the crystal lattice of minerals or in organic matter that is difficult to decompose (hardly available)
Nutrients can be absorbed in different ways. One possibility is the root suction. The negative pressure in the root area, which results from the evaporation of water from the leaves, means that the plant sucks the soil water to the root hairs. The ions dissolved in the water are sucked in as well. The root suction reaches a few centimeters and only captures freely moving ions. The amount absorbed depends on the concentration of ions in the soil water and the water consumption of the plant. The soil solution in the immediate root area is depleted by the ion uptake by the plant.
This results in the so-called diffusion. Since the ion concentration is much higher further away from the root area, the ion distribution is evened out as the ions or molecules migrate in the direction of the root. This balances out the concentrations. Water is used as the transport and solvent. For this reason, nutrient deficiency symptoms often occur during drought.
Root growth can also promote nutrient uptake. Root tips that come into direct contact with exchangers can literally "graze" on them. The root grows towards the exchangers. The growth rhythm, which is different for each plant species, means that the roots are not always equally active. If you know about the root activity of a plant, this knowledge can be used to choose the best time for fertilization. In times of high root activity, nutrient uptake is particularly good. This saves on fertilization costs and reduces the risk of leaching.
Processes in the root
A prerequisite for the water absorption of the roots is that the salt concentration in the root cells is higher than in the soil water. The nutrient salts must therefore migrate to an area with a high salt concentration when they are absorbed. Since this is not possible, the ions have to be brought into the root hair cells against a concentration gradient, using energy. Since the cell membrane is impenetrable for the ions, they are transported through by special carriers and thus reach the inside of the cell.
The energy for these processes is generated by root breathing. This is also the reason why plants often show symptoms of nutrient deficiency when waterlogged. Loosening the soil helps against this, as it promotes gas exchange and thus leads to increased root respiration and increased nutrient uptake. The root should also be sufficiently supplied with reserve substances.
To a limited extent, the plant can exclude nutrient salt ions from uptake. This is due to the fact that certain carriers are available for certain ions, which are based on the electrical charge and the ion diameter. The potassium and ammonium ions have the same charge and roughly the same diameter. Because of this, they compete for the same carrier.
If there is an oversupply of one of the two ions, the other is absorbed in smaller quantities. This phenomenon can lead to a nutrient deficiency and is called antagonism. This phenomenon also occurs, for example, in soils or substrates that are well supplied with lime. Due to their high concentration in the soil solution, the calcium ions accumulate there at the roots and inhibit the absorption of other ions, such as magnesium and potassium ions.
Pasture water, the indole-3-butyric acid it contains stimulates root formation.
Exchanges
Anions, such as SO42- and NO3-, are usually found freely in the soil solution. Cations, on the other hand, are bound to clay and humus particles. They can only be taken up by the plant if it displaces them from the exchangers by releasing H+ ions. The H+ ions are a waste product of root respiration. With the help of the H+ ions, the plant can release nutrients bound to exchangers and, to a limited extent, reserve nutrients.
The emission of HCO3- ions also has a reason. In order to maintain the prevailing electrical charge in the plant cells even when ions are taken up, one HCO3- ion must be released for each anion and one H+ ion for each cation.
Plants also secrete other compounds that can be used to make nutrient salts available. These include, for example, the so-called chelates, which release fixed ions and release them to the plant roots.
Changes in soil response to nutrient salt uptake
If the cation of a mineral fertilizer salt is absorbed by the plant more quickly than the anion, it releases more H+ ions to balance the charge. This lowers the pH of the soil solution. In this case, it is a physiologically acidic fertilizer. This reaction usually occurs when the cation is monovalent and the accompanying anion is divalent. Typical examples are potassium sulphate (K2SO4) and sulfuric acid ammonia ((NH4)2SO4). In addition, with sulfuric acid ammonia, the ammonium ions (NH4+) not directly absorbed by the plant are converted to nitrate (NO3-) in the soil. Here, H+ ions are released again.
A preferential uptake of anions, on the other hand, leads to an increased release of HCO3- ions. This raises the pH value. In this case one speaks of physiologically alkaline fertilizers. Sodium nitrate (NaNO3) and nitrate of lime (Ca(NO3)2) are examples of this.
Each mineral fertilizer has a certain influence on the soil reaction. When fertilizing with sulfuric acid ammonia, the H+ ions released cause a loss of lime. This side effect is undesirable and must later be compensated for by appropriate liming. Nevertheless, this acid surge also has the advantage that trace nutrients become more readily available.
Water:
Water intake is critical for maximum growth. Plants absorb nutrients in the form of moisture through their roots. The more roots and fine roots, the better. It is extremely important to irrigate with high-quality water, it must be low in salt, so do not use tap water for irrigation. Rainwater or water from reverse osmosis are more suitable. Many types of root rot can be prevented with proper drainage. Therefore, it is important to test soil and irrigation water. Make sure that the water can drain off easily and that the soil mixture in the pot has a drainage layer and the soil is permeable to air.
Nitrification and denitrification are points that I will go into more intensively later. The root can only absorb nutrient salts as ions dissolved in the water. For this reason, the soil should be sufficiently moist to allow the uptake of mineral fertilizers and compounds mineralized by soil creatures. The water in the soil splits the fertilizer salts into ions.
By watering the plants with osmosis water, they can thrive better because they get the acidic substrate they need to grow. The water is freed from all coarse pollutants with the help of the water filter upstream of the membrane.
Essentially, reverse osmosis water has gone through a high-pressure filtration process that removes 95–99% of dissolved salts and impurities. This is accomplished by forcing water through a semi-permeable membrane that acts as a filter. The significant pressure exerted allows pure water to flow through while the minerals, salts and contaminants remain on the other side of the membrane.
Light & Photosynthesis:
Photosynthesis is the process where the energy of light is converted into glucose, i.e. types of sugar. By consuming sugars, plants grow. There are causal relationships between root growth and light.
The photosynthetic photon flux density (PPFD for short) is measured with the number of photons in the range of 400 - 700 nm that hit a surface in a certain period of time. In 2009, University of Mississippi scientist Suman Chandra examined three photosynthetic determinants (PPFD, temperature, and CO 2 concentrations) for their effect on the rate of photosynthesis in cannabis.
The center around 480nm is enormously important, the phytochrome is the sensor, the leaves are the power plants, the roots are the lines, but the leaves in particular have additional functions where a broad spectrum is simply important.
He came to the conclusion that at temperatures of 20 to 25 °C an increase in PPFD also led to an increased rate of photosynthesis. The maximum rate of photosynthesis was at 30°C and a PPFD of 1500 µmol m-2s-1. With a higher PPFD, the rate of photosynthesis decreased again.
An increase in CO 2 concentrations also had a positive effect on plant growth. Chandra concluded that the optimal comfort zone for cannabis plants is between 25 and 30°C in combination with light sources with a PPFD of around 1500 µmol m-2s-1.
But, photosynthesis doesn't start at 400nm and stop at 700nm as marketing and paid researchers like to tell us!
Nichia Horticulture Array - 2017
400nm - 700nm = 319,025 μmol/s
380nm - 780nm = 335,920 μmol/s
DLI of Array = 5,576 mol/m2/day
Oxygen and Carbon Dioxide:
CO² is the chemical molecular formula for the molecule carbon dioxide, also known as carbon dioxide, which consists of carbon and oxygen. Carbon dioxide gas is colourless, soluble in water, non-flammable, odorless and non-toxic. Plants need CO2 to produce glucose, and there is enough of that in the atmosphere. Plants need oxygen to breathe. Overwatering depletes the soil of oxygen, leading to root rot, and the processes that lead to root rot are processes that cause blockages in nutrient uptake.
Temperature:
Temperature has a major impact on growth rate. If the temperature is too high (above 30°C), photosynthesis decreases, while if the temperature is too low, growth, fruiting and flowering slow down. The ideal soil temperature should be around 20°C to 24°C. There are processes in the soil that below 18C° can trigger stagnation in nutrient uptake. Soil temperature and leaf (stomata) temperature are separate, but both are important factors in growing cannabis.
Temperature and humidity have effects that can add up!
The stomata are specialized cells in the leaves that open and close. In this way, they limit the amount of water vapor that can evaporate. As the temperature rises, the stomata allow more evaporation because they open. It is difficult to measure the opening of the stomata.
Humidity:
If humidity is too low or too high, it can stunt plant growth. When the humidity is too high, plants' ability to evaporate water through the leaves is impaired, resulting in reduced uptake of water and nutrients through the roots. It also increases the likelihood of fungus taking hold. If the humidity is too low, your plant may show signs of scorching, which can lead to a loss of chlorophyll.
Nutrient/water uptake and transport
The roots of a plant are connected to the shoot axis and the leaves by a system of tubing. This conduction system consists of vascular bundles that run through the entire plant.
Vascular bundles consist of either xylem or phloem, or both together in various configurations. The main elements in the xylem are the so-called tracheids, together with the so-called tracheae in the covered plant families. Tracheae are elongated, dead cells that are lined with a special substance (lignin) for reinforcement. The partition walls between the individual cells have been dissolved, creating a coherent system of lines. The tracheae are accompanied by the tracheids. Their peculiarity is that they are connected to each other by perforated walls (so-called pits). Tracheae and tracheids are found inside a stem and form the xylem or wood. Gymnosperms only have tracheids as a conduction system, angiosperms have both cell types.
Unlike the xylem, the phloem consists of living cells. These are the sieve elements such as sieve tubes and sieve cells. Sieve tubes are incompletely formed cells that are alive but lack important components of a living cell, such as a cell nucleus or a vacuole. They are therefore only viable in connection with the neighboring companion cells. Companion cells have the function of loading the sieve tubes with assimilates (sugars and amino acids). In contrast to the sieve tubes, they have all the necessary components of a cell and probably arose together with the sieve tubes from the incomplete division of a cell. The sieve tubes and their companion cells are found only in angiosperms. Sieve cells are only found in gymnosperms. They have remnants of a cell nucleus, other cell components such as the vacuole are missing. They are also connected to one another by perforated cell walls (screen panels). The cell walls lie at an angle to one another.
At the upper end of the pathways are the leaves. They have small openings (stomata, or stomata) that they can open and close "as needed." Gas exchange takes place via these stomata: carbon dioxide diffuses into the plant (important for photosynthesis) and water vapor diffuses out (important for cooling the leaves, among other things). Occasionally, water is also actively released in the form of drops (guttation). The water escaping at the top of the leaves creates a suction that draws water up the stem of the plant. This creates a negative pressure in the plant and also in the roots. Consequence: Water is "drawn" from the roots upwards and from the soil regions into the plant (transpiration suction, negative water potential).
Another trick is the accumulation of substances (e.g. polysaccharides such as starch) in the root cells. This creates a higher concentration of sugar in the root cells than outside and in the course of the diffusion equilibrium, water flows into the cell (osmosis, root pressure).
With the water, dissolved nutrients also get into the root cells, from where they are transported further. In order to get as many nutrients as possible from the environment, the fine roots release protons (hydrogen ions, simply positively charged) into the soil water (so-called acidification). The protons displace the nutrients (nitrogen in the form of ammonium (NH3) or nitrate (NO3-), calcium (Ca2), magnesium (Mg2), phosphorus in the form of phosphate (PO4-), potassium (K), sodium (Na ) and sulfur (S2) from soil particles (so-called exchangers such as clay minerals, humic substances), so that the nutrients dissolve and are absorbed with the soil water.
Home remedies
Improve root growth in general and for cuttings. Yeast, dissolve 100 g of dry yeast in a liter of lukewarm water. Put cuttings in leave for a day, then rinse thoroughly.
Aloe vera juice, put 1 tablespoon of aloe vera gel in a glass of water. Root shoots appear after a week.
Sources and literature:
Hortipendium (German Language)
Plant research (German Language)
Research on cannabis
LED Inside Nichia
There are 6 important factors that can affect cannabis growth and flowering: nutrients, water supply, light intensity, oxygen and CO².
Nutrients:
Most of the building blocks of plants are present in the atmosphere. Fertilization affects growth by only about 6 percent. Therefore, do not use more fertilizer than necessary, as this could affect growth. The root can only absorb nutrient salts as ions dissolved in the water. For this reason, the soil should be sufficiently moist to allow the uptake of mineral fertilizers and compounds mineralized by soil creatures. The water in the soil splits the fertilizer salts into ions. For example, nitrate of lime (Ca(NO3)2) breaks down into the positively charged calcium ion (Ca2+) and two negatively charged nitrate ions (NO3-).
The nutrient salt ions are found in soil in three forms:
- as freely mobile ions dissolved in the water (very easily absorbable)
- interchangeably bound to negatively charged clay and humus particles (relatively easy to absorb)
- as reserve nutrients in the crystal lattice of minerals or in organic matter that is difficult to decompose (hardly available)
Nutrients can be absorbed in different ways. One possibility is the root suction. The negative pressure in the root area, which results from the evaporation of water from the leaves, means that the plant sucks the soil water to the root hairs. The ions dissolved in the water are sucked in as well. The root suction reaches a few centimeters and only captures freely moving ions. The amount absorbed depends on the concentration of ions in the soil water and the water consumption of the plant. The soil solution in the immediate root area is depleted by the ion uptake by the plant.
This results in the so-called diffusion. Since the ion concentration is much higher further away from the root area, the ion distribution is evened out as the ions or molecules migrate in the direction of the root. This balances out the concentrations. Water is used as the transport and solvent. For this reason, nutrient deficiency symptoms often occur during drought.
Root growth can also promote nutrient uptake. Root tips that come into direct contact with exchangers can literally "graze" on them. The root grows towards the exchangers. The growth rhythm, which is different for each plant species, means that the roots are not always equally active. If you know about the root activity of a plant, this knowledge can be used to choose the best time for fertilization. In times of high root activity, nutrient uptake is particularly good. This saves on fertilization costs and reduces the risk of leaching.
Processes in the root
A prerequisite for the water absorption of the roots is that the salt concentration in the root cells is higher than in the soil water. The nutrient salts must therefore migrate to an area with a high salt concentration when they are absorbed. Since this is not possible, the ions have to be brought into the root hair cells against a concentration gradient, using energy. Since the cell membrane is impenetrable for the ions, they are transported through by special carriers and thus reach the inside of the cell.
The energy for these processes is generated by root breathing. This is also the reason why plants often show symptoms of nutrient deficiency when waterlogged. Loosening the soil helps against this, as it promotes gas exchange and thus leads to increased root respiration and increased nutrient uptake. The root should also be sufficiently supplied with reserve substances.
To a limited extent, the plant can exclude nutrient salt ions from uptake. This is due to the fact that certain carriers are available for certain ions, which are based on the electrical charge and the ion diameter. The potassium and ammonium ions have the same charge and roughly the same diameter. Because of this, they compete for the same carrier.
If there is an oversupply of one of the two ions, the other is absorbed in smaller quantities. This phenomenon can lead to a nutrient deficiency and is called antagonism. This phenomenon also occurs, for example, in soils or substrates that are well supplied with lime. Due to their high concentration in the soil solution, the calcium ions accumulate there at the roots and inhibit the absorption of other ions, such as magnesium and potassium ions.
Pasture water, the indole-3-butyric acid it contains stimulates root formation.
Exchanges
Anions, such as SO42- and NO3-, are usually found freely in the soil solution. Cations, on the other hand, are bound to clay and humus particles. They can only be taken up by the plant if it displaces them from the exchangers by releasing H+ ions. The H+ ions are a waste product of root respiration. With the help of the H+ ions, the plant can release nutrients bound to exchangers and, to a limited extent, reserve nutrients.
The emission of HCO3- ions also has a reason. In order to maintain the prevailing electrical charge in the plant cells even when ions are taken up, one HCO3- ion must be released for each anion and one H+ ion for each cation.
Plants also secrete other compounds that can be used to make nutrient salts available. These include, for example, the so-called chelates, which release fixed ions and release them to the plant roots.
Changes in soil response to nutrient salt uptake
If the cation of a mineral fertilizer salt is absorbed by the plant more quickly than the anion, it releases more H+ ions to balance the charge. This lowers the pH of the soil solution. In this case, it is a physiologically acidic fertilizer. This reaction usually occurs when the cation is monovalent and the accompanying anion is divalent. Typical examples are potassium sulphate (K2SO4) and sulfuric acid ammonia ((NH4)2SO4). In addition, with sulfuric acid ammonia, the ammonium ions (NH4+) not directly absorbed by the plant are converted to nitrate (NO3-) in the soil. Here, H+ ions are released again.
A preferential uptake of anions, on the other hand, leads to an increased release of HCO3- ions. This raises the pH value. In this case one speaks of physiologically alkaline fertilizers. Sodium nitrate (NaNO3) and nitrate of lime (Ca(NO3)2) are examples of this.
Each mineral fertilizer has a certain influence on the soil reaction. When fertilizing with sulfuric acid ammonia, the H+ ions released cause a loss of lime. This side effect is undesirable and must later be compensated for by appropriate liming. Nevertheless, this acid surge also has the advantage that trace nutrients become more readily available.
Water:
Water intake is critical for maximum growth. Plants absorb nutrients in the form of moisture through their roots. The more roots and fine roots, the better. It is extremely important to irrigate with high-quality water, it must be low in salt, so do not use tap water for irrigation. Rainwater or water from reverse osmosis are more suitable. Many types of root rot can be prevented with proper drainage. Therefore, it is important to test soil and irrigation water. Make sure that the water can drain off easily and that the soil mixture in the pot has a drainage layer and the soil is permeable to air.
Nitrification and denitrification are points that I will go into more intensively later. The root can only absorb nutrient salts as ions dissolved in the water. For this reason, the soil should be sufficiently moist to allow the uptake of mineral fertilizers and compounds mineralized by soil creatures. The water in the soil splits the fertilizer salts into ions.
By watering the plants with osmosis water, they can thrive better because they get the acidic substrate they need to grow. The water is freed from all coarse pollutants with the help of the water filter upstream of the membrane.
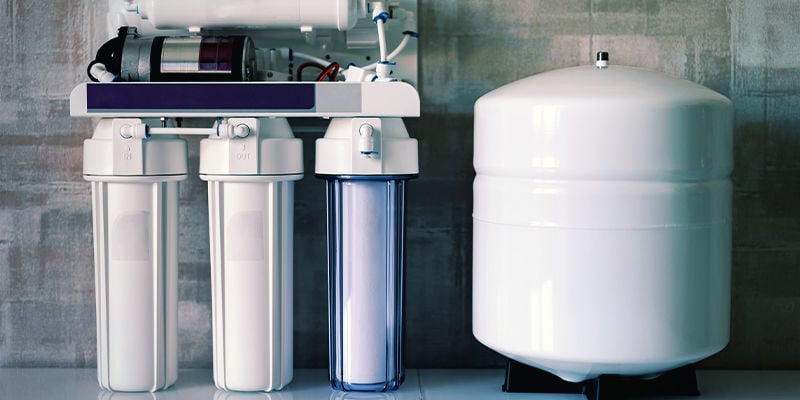
Essentially, reverse osmosis water has gone through a high-pressure filtration process that removes 95–99% of dissolved salts and impurities. This is accomplished by forcing water through a semi-permeable membrane that acts as a filter. The significant pressure exerted allows pure water to flow through while the minerals, salts and contaminants remain on the other side of the membrane.
Light & Photosynthesis:
Photosynthesis is the process where the energy of light is converted into glucose, i.e. types of sugar. By consuming sugars, plants grow. There are causal relationships between root growth and light.
The photosynthetic photon flux density (PPFD for short) is measured with the number of photons in the range of 400 - 700 nm that hit a surface in a certain period of time. In 2009, University of Mississippi scientist Suman Chandra examined three photosynthetic determinants (PPFD, temperature, and CO 2 concentrations) for their effect on the rate of photosynthesis in cannabis.
The center around 480nm is enormously important, the phytochrome is the sensor, the leaves are the power plants, the roots are the lines, but the leaves in particular have additional functions where a broad spectrum is simply important.
He came to the conclusion that at temperatures of 20 to 25 °C an increase in PPFD also led to an increased rate of photosynthesis. The maximum rate of photosynthesis was at 30°C and a PPFD of 1500 µmol m-2s-1. With a higher PPFD, the rate of photosynthesis decreased again.
An increase in CO 2 concentrations also had a positive effect on plant growth. Chandra concluded that the optimal comfort zone for cannabis plants is between 25 and 30°C in combination with light sources with a PPFD of around 1500 µmol m-2s-1.
But, photosynthesis doesn't start at 400nm and stop at 700nm as marketing and paid researchers like to tell us!
Nichia Horticulture Array - 2017
400nm - 700nm = 319,025 μmol/s
380nm - 780nm = 335,920 μmol/s
DLI of Array = 5,576 mol/m2/day
Oxygen and Carbon Dioxide:
CO² is the chemical molecular formula for the molecule carbon dioxide, also known as carbon dioxide, which consists of carbon and oxygen. Carbon dioxide gas is colourless, soluble in water, non-flammable, odorless and non-toxic. Plants need CO2 to produce glucose, and there is enough of that in the atmosphere. Plants need oxygen to breathe. Overwatering depletes the soil of oxygen, leading to root rot, and the processes that lead to root rot are processes that cause blockages in nutrient uptake.
Temperature:
Temperature has a major impact on growth rate. If the temperature is too high (above 30°C), photosynthesis decreases, while if the temperature is too low, growth, fruiting and flowering slow down. The ideal soil temperature should be around 20°C to 24°C. There are processes in the soil that below 18C° can trigger stagnation in nutrient uptake. Soil temperature and leaf (stomata) temperature are separate, but both are important factors in growing cannabis.
Temperature and humidity have effects that can add up!
The stomata are specialized cells in the leaves that open and close. In this way, they limit the amount of water vapor that can evaporate. As the temperature rises, the stomata allow more evaporation because they open. It is difficult to measure the opening of the stomata.

Humidity:
If humidity is too low or too high, it can stunt plant growth. When the humidity is too high, plants' ability to evaporate water through the leaves is impaired, resulting in reduced uptake of water and nutrients through the roots. It also increases the likelihood of fungus taking hold. If the humidity is too low, your plant may show signs of scorching, which can lead to a loss of chlorophyll.
Nutrient/water uptake and transport
The roots of a plant are connected to the shoot axis and the leaves by a system of tubing. This conduction system consists of vascular bundles that run through the entire plant.
Vascular bundles consist of either xylem or phloem, or both together in various configurations. The main elements in the xylem are the so-called tracheids, together with the so-called tracheae in the covered plant families. Tracheae are elongated, dead cells that are lined with a special substance (lignin) for reinforcement. The partition walls between the individual cells have been dissolved, creating a coherent system of lines. The tracheae are accompanied by the tracheids. Their peculiarity is that they are connected to each other by perforated walls (so-called pits). Tracheae and tracheids are found inside a stem and form the xylem or wood. Gymnosperms only have tracheids as a conduction system, angiosperms have both cell types.
Unlike the xylem, the phloem consists of living cells. These are the sieve elements such as sieve tubes and sieve cells. Sieve tubes are incompletely formed cells that are alive but lack important components of a living cell, such as a cell nucleus or a vacuole. They are therefore only viable in connection with the neighboring companion cells. Companion cells have the function of loading the sieve tubes with assimilates (sugars and amino acids). In contrast to the sieve tubes, they have all the necessary components of a cell and probably arose together with the sieve tubes from the incomplete division of a cell. The sieve tubes and their companion cells are found only in angiosperms. Sieve cells are only found in gymnosperms. They have remnants of a cell nucleus, other cell components such as the vacuole are missing. They are also connected to one another by perforated cell walls (screen panels). The cell walls lie at an angle to one another.
At the upper end of the pathways are the leaves. They have small openings (stomata, or stomata) that they can open and close "as needed." Gas exchange takes place via these stomata: carbon dioxide diffuses into the plant (important for photosynthesis) and water vapor diffuses out (important for cooling the leaves, among other things). Occasionally, water is also actively released in the form of drops (guttation). The water escaping at the top of the leaves creates a suction that draws water up the stem of the plant. This creates a negative pressure in the plant and also in the roots. Consequence: Water is "drawn" from the roots upwards and from the soil regions into the plant (transpiration suction, negative water potential).
Another trick is the accumulation of substances (e.g. polysaccharides such as starch) in the root cells. This creates a higher concentration of sugar in the root cells than outside and in the course of the diffusion equilibrium, water flows into the cell (osmosis, root pressure).
With the water, dissolved nutrients also get into the root cells, from where they are transported further. In order to get as many nutrients as possible from the environment, the fine roots release protons (hydrogen ions, simply positively charged) into the soil water (so-called acidification). The protons displace the nutrients (nitrogen in the form of ammonium (NH3) or nitrate (NO3-), calcium (Ca2), magnesium (Mg2), phosphorus in the form of phosphate (PO4-), potassium (K), sodium (Na ) and sulfur (S2) from soil particles (so-called exchangers such as clay minerals, humic substances), so that the nutrients dissolve and are absorbed with the soil water.
Home remedies
Improve root growth in general and for cuttings. Yeast, dissolve 100 g of dry yeast in a liter of lukewarm water. Put cuttings in leave for a day, then rinse thoroughly.
Aloe vera juice, put 1 tablespoon of aloe vera gel in a glass of water. Root shoots appear after a week.
Sources and literature:
Hortipendium (German Language)
Plant research (German Language)
Research on cannabis
LED Inside Nichia
Last edited: